Turbulent Kinetic Energy and Budget of Heterogeneous Open Channel with Gravel and Vegetated Beds
Author(s): OP Folorunso
Abstract
Turbulent kinetic energy (TKE) and budget are indispensable hydraulic parameters to determine turbulent scales and processes resulting from various and different natural hydraulic features in open channels. This paper focuses on experimental investigation of turbulent kinetic energy and budget in a heterogeneous open channel flow with gravel and vegetated beds. Results indicate the turbulent kinetic energy (TKE) value over gravel region of the heterogeneous bed remains approximately constant with flow depth. The highest turbulent kinetic energy was calculated for flexible vegetation arrangement compared to the rigid vegetation. The estimation of the turbulent kinetic energy budget shows the higher values of turbulence production recorded over the flexible vegetated bed, consequently, the dissipation rate exhibits faster decay of turbulence kinetic energy over the vegetated bed in comparison to the gravel bed.
Introduction
The modified turbulent kinetic energy (K) in vegetated flow
assumed to be steady can be expressed as [1]:
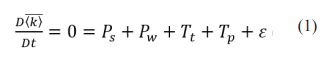
where Ps, is the shear production, Pw, the wake production, Tt
,
turbulent transport, Tp
, pressure transport, and ε the dissipation.
In a fully developed flow, the largest terms are the shear turbulent
production Ps
and dissipation ε. Under equilibrium conditions,
these terms tends to be in balance and under non-equilibrium
conditions, turbulent kinetic energy is transported either through
turbulent transport Tt
, or pressure transport Tp
. Example of
turbulent kinetic energy budget is showing in Figure 1 [1].
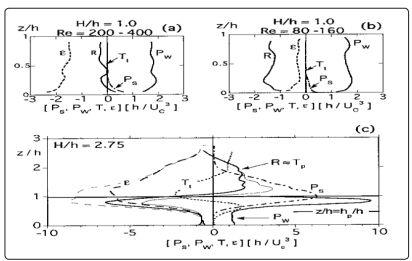
Figure 1: Turbulent Kinetic Energy for Emergent and Submerged
Conditions [1].
Turbulent Intensity and Kinetic Energy
The magnitude of turbulence can be quantified using the turbulence
intensity, i.e,

Where σ is the standard deviation of the velocity fluctuation.
Experimental observations in open channel flows have shown that
the turbulence intensity will be greater near the channel boundary
where turbulence is being generated, and decrease with depth
away from the boundary towards the free surface [2]
Turbulence Kinetic Energy (K)
With turbulence being transferred by the turbulent kinetic energy
(K), the K relates the mean kinetic energy per unit mass of fluid
with the turbulent eddies; this is characterized by the three
dimensional turbulence intensities. The turbulent kinetic energy

was obtained on the basis of the turbulence
intensity values along the streamwise, lateral, and vertical
directions.
Material and Methods
The experiments were conducted in 22mm long rectangular
re-circulating flume of width B=614mm at the University of
Birmingham. The channel is supplied from a constant head
tank with a capacity of 45,500l in the laboratory roof. A flow
discharges (Q) (30.0 l/s) with corresponding flow depth (H) of
130mm width to depth ratio (B / H) of 4.7 achieve subcritical flow
condition was investigated. In what follows these experimental
conditions are referred to as EXPT1 and EXPT2 respective
Detailed velocity measurements were made at three cross sections
(CRS1, CRS2 and CRS3) at distances of 17.5m, 17.85m and 18.2m
respectively downstream from the channel inlet. In the results that
follow, the gravel region of the bed extends over (0≤y/B≤0.5), the
interface occurs at (y/
B=0.5), and the vegetated region extends
over (0.5≤y/
B≤1.0), wherey is the lateral distance from the left
hand side looking downstream and B is the channel width. The
streamwise direction x is in the direction of flow. The transverse
direction y is perpendicular to x in the lateral direction, while
the vertical direction is denoted by z and is perpendicular to the
xy plane (positive upwards). The corresponding time average
velocity components are U,V,W respectively. Figure 2 shows the
bed configuration for EXPT1 and EXPT2 [4-10].
Figure 2: Bed Configuration for EXPT1 and EXPT2
Results and Discussion
Figures 3 and 4 show the lateral distribution of the turbulent
kinetic energy for CRS3. Figure 3 shows that the maximum
value of turbulent kinetic energy (K) occurs near bed (z/
H≤0.2)
over the vegetated zone. For depths greater thanz/
H≈0.2, the
turbulent kinetic energy value reduces towards the free surface.
However, the TKE value over gravel region in EXPT2 remains
approximately constant with flow depth (Figure 4). The highest
turbulent kinetic energy was calculated for flexible vegetation
arrangement compared to the rigid vegetation [11-20].
Figure 3: Lateral Disribution of Turbulent Kinetic Energy (K)
at CRS3 EXPT1
Figure 4: Lateral Disribution of Turbulent Kinetic Energy (K)
CRS3 (bottom) EXPT2
Turbulent Energy Budget
To clarify further the structure of turbulence in the flow, the
turbulent energy terms were explored using the velocity data to
explain the relative significance of the processes that control the
turbulent flow. The turbulence kinetic energy was obtained as
described in the introduction, the vertical turbulence transport

viscosity [3]. Figure 5 and Figure 6 show the turbulence terms for
the flow, with the values normalized by the depth of flow. Both the
turbulence production and the turbulence kinetic energy attained
maxima near the bed, and decrease towards the free surface (Figure
5 and Figure 6). In contrast, the turbulence transport Tr
tends to
increase towards the free surface when compared with other
turbulence terms; this indicates the transport of the near bed
turbulent energy towards the free surface. Similar mechanisms
have been observed by others [3]. Hence, in this region, Tr serves
as source of turbulence compensating for reduced turbulence
production at the free surface. However, it should be noted that
the high turbulence intensity near the bed over the vegetated
region (Result not presented) in EXPT1 indicates an inflection
point; this becomes an essential source of turbulence generation
hence enhancing turbulence production and kinetic energy over
the vegetated bed relative to gravel bed. It can be observed that
the higher values of turbulence production are recorded over the
vegetated bed in EXPT1 (Figure 5b). However, comparison with
turbulent intensity shows that this is enhanced by the vertical
velocity fluctuating component of the flow. Turbulence production
over the vegetated bed in EXPT2 is comparable to the turbulence
production over the gravel bed with similar magnitude of
production terms (Figure 6a and 6b). The dissipation rate exhibits
faster decay of turbulence kinetic energy over the vegetated bed
in comparison to the gravel bed (Figure5and6) [21-40].

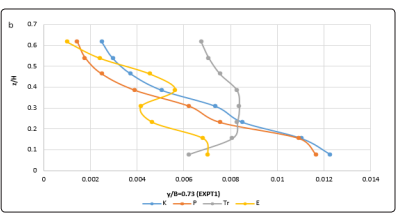
Figure 5: Vertical Distribution of the Turbulent Energy Terms
(EXPT1): (a): Gravel Bed (b): Vegetated Bed
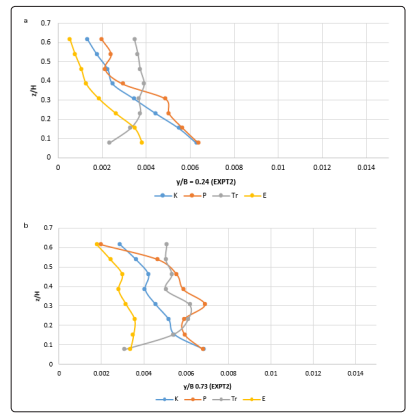
Figure 6: Vertical Distribution of the Turbulent Energy Terms
(EXPT2): (a): Gravel Bed (b): Vegetated Bed
Conclusion
It is concluded from the current research work that the maximum
turbulence production and kinetic energy attained maxima near the
bed due to shear, and decreases towards the free surface. However,
the near bed turbulent energy is transported towards the free
surface hence tends to increase turbulent transport Tr
towards the
free surface when compared with other turbulence terms [41-51].
References
- Nepf HM, Vivoni ER (2000) Flow structure in depth-limited,
vegetated flow. Journal of Geophysical Research 105: 28,547-
28,557.
- Carollo FG, Ferro V, Termini D (2005) Analyzing turbulence
intensity in gravel bed channels. Journal of Hydraulic
Engineering 131: 1050-1061.
- Nezu I, Nakagawa H (1993) Turbulence in open-channel
flows. Rotterdam, A.A. Balkema.
- Anderson J (1995) Introduction to Computational Fluid
Dynamics. McGraw Hill, Edition 6 https://www.mathematik.
uni-dortmund.de/~kuzmin/cfdintro/lecture1.pdf.
- Cea L, Puertas J, Pena L (2007) Velocity measurements on
highly turbulent free surface flow using ADV. Experiments
in Fluids 42: 333-348.
- Cheng NS, Nguyen HT, Tan SK, Shao S (2012) Scaling of
velocity profiles for depth-limited open channel flows over
simulated rigid vegetation. Journal of Hydraulic Engineering
138: 673-683.
- Davidson PA (2004) Turbulence - an introduction for scientists
and engineers. Oxford University Press.
- Finnigan J (2000) Turbulence in plant canopies. Journal of
Fluid Mechanics 32: 519-571.
- Ghisalberti M, Nepf HM (2004) The limited growth of
vegetated shear layers. Water Resource. Res 40: 1-12.
- Ghisalberti M, Nepf H (2002) Mixing layers and coherent
structures in vegetated aquatic flow. Journal of Geophysical
Research-Earth Surface 107: 527-551.
- Hinze JO (1975) Turbulence. McGraw-Hill series in
mechanical engineering.
- Ikeda SMKK, Ohta (1995) Flow over flexible vegetation and
3-D structure of organized vortex associated with honami.
Journal of Hydraulic, Coastal and Environmental Enginerring
515: 33-43.
- Ishigaki, T., Muto, Y. & Shiono, K. 2010. Flow structure and
resistance in compound open channel. Nagare, 29, 177-186.
- Jesson, M., Sterling, M. & Bridgeman, J. 2010. Turbulent
structures in heterogenous channels and their effects
on conyeyance. First IAHR European Div. Congress.,
International Association for Hydro-Environment Engineering
and Research (IAHR), Madrid, Spain.
- Jesson M, Sterling M, Brigdeman J (2013) Modelling flow in
an open channel with heterogeneous bed roughness. Journal
of Hydraulic Engineering 139: 195-204.
- Jesson M (2011) The effect of heterogeneous roughness on
conveyance capacity and application of the Shiono-Knight
Method Method. PhD, University of Birmingham.
- Jesson M, Sterling M, Bridgeman J (2012) An experimental
study of turbulence in a heterogeneous channel. Water
Management Proceedings of the Institution of Civil Engineers
166: 16-26.
- Jimenez J (2004) Turbulent flows over rough walls. Fluid
Mech 36: 173-96.
- Kouwen N, Unny TE (1980) Flexible roughness in open
channels. Journal of Hydraulic Engineering-Asce 99: 713-
727.
- Lohrmann A, Cabrera R, Kraus NC (1994) Acoustic Doppler
velocimeter (ADV) for Laboratory Use. Fundamentals
and Advancements in Hydraulic Measurements and
Experimentation. Buffalo, New York. ASCE 351-365.
- Luhar M, Rominger J, Nepf H (2008) Interaction between
flow, transport and vegetation spatial structure. Environmental
Fluid Mechanics 8: 423-439.
- Manes C, Pokrajac D, Mcewan I, Nikora V (2009) Turbulence
structure of open channel flows over permeable and
impermeable beds: A comparative study. Physics of Fluids
21: 125109(1-12).
- Nakagawa H, Nezu I, Ueda H (1975) Turbulence of Open
channel flow over smooth and rough beds. Proceedings of
JSCE 241: 155-168.
- Naot D, Rodi W (1981) Calculation of secondary currents in
channel flow. ASCE, JHydraulic s Div 108: 948-68.
- Nepf HM (2012) Hydrodynamics of vegetated channels.
Journal of Hydraulic Research 50: 262-279.
- Nepf H, Ghisalberti M (2008) Flow and transport in channels
with submerged vegetation. . Acta Geophysical 56: 753-777.
- Nepf HM (2004) Vegetated flow dynamics. Coastal and
Estuarine Studies 59: 137-163.
- Nezu I, Sanjou M (2008) Turburence structure and coherent
motion in vegetated canopy open-channel flows. Journal of
Hydro-Environment Research 2: 62-90.
- Nezu I, Sanjou M, Okamoto T (2006) Turbulent structure anddispersive properties in vegetated canopy open-channel flows.
- Nezu I, Onitsuka K (2001) Turbulent structures in
partly vegetated open-channel flows with LDA and PIV
measurements. Journal of Hydraulic Research 39: 629-642.
- Nezu I, Tominaga A, Nakagawa H (1993) Field measurements
of secondary currents in straight rivers. Journal of Hydraulic
Engineering-Asce 119: 598-614.
- Nezu I, Rodi W (1986) Open channel measurements with a
Laser Doppler Anemometer. Journal of Hydraulic Engineering
112: 335-355.
- Nezu I (1977) Turbulence intensities in open-channel flows.
Journal of JSCE 261: 67-76.
- Nikora N, Nikora V (2010) Flow penetration into the canopy
of the submerged vegetation: definitions and quantitative
estimates. River Flow 2010 - Dittrich, Koll, Aberle &
Geisenhainer 438-444.
- Nikora V (2009) Hydrodynamics of aquatic ecosystems: an
interface between ecology, biomechanics and enviromental
fluid mechanics. River Research and Applications 26: 367-
384.
- Nikora VI, Goring DG, Mcewan I, Griffiths G (2001)
Spatially-averaged open-channel flow over a rough bed.
Hydraulic Engineering 127: 123-133.
- Nikora VI, Goring DG (1998) ADV Measurements of
turbulence: can we improve their interpretation. Journal of
Hydraulic Engineering 124: 630-634.
- Okamoto T, Nezu I (2009) Turbulence Structure and Monami
Phenomena in Flexible Vegetated Open Channel Flows.
Journal of Hydraulic Engineering 47: 798-810.
- Peltier Y, Proust S, Riviere N, Paquier A, Shiono K (2013)
Turbulent flows in straight compound open-channel with
a transverse embankment on the floodplain. Journal of
Hydraulic Research, 0022-1686.
- Raupach M, Finingan J, Brunet Y (1996) Coherent Eddies
and Turbulence in Vegetation Canopies: The Mixing Later
Analogy. . Boundary Layer Meteorology 60: 375-395.
- Raupach M, Shaw R (1981) Turbulence In and Above Plant
Canopies Annual Review of Fluid Mechanics 13: 97-129.
- Shimizu Y, Tsujimoto T (1993) Comparison of flood flow
structure between compound channel and channel with
vegetation zone. Proceedings of 25th IAHR Congress, Tokyo
1 A-3-4: 97-104.
- Shiono K, Takeda M, Yang K, Sugihara Y, Ishigaki T (2012)
Modeling of vegetated rivers for inbank and overbank flows.
Proceeding of the International Conference on Fluvial
Hydraulics - River Flow 1: 263-269.
- Shiono K, Ishigaki T, Kawanaka R, Heatlie (2009) Influence
of one line vegetation on stagedischarge rating curves in
compound channel. 33rd IAHR Congress, Water Engineering
for a sustainable Environment 1475-1482.
- Shiono K, Muto Y (1998) Complex flow mechanisms in
compound meandering channels with overbank flow. Journal
of Fluid Mechanics 376: 221-261.
- Shiono K, Knight DW (1991) Turbulent open-channel flows
with variable depth across the channel. J. Fluid Mech 222:
617-646.
- Suncan (2012) Introduction to compound channel flow
analysis for floodplains. SunCam online.
- Sun X, Shiono K (2010) Flow characteristics in vegetated
compound channel. Enviromental Hydraulics 233-239.
- Tsujimoto T (1999) Fluvial processes in streams with
vegetation. Journal of Hydraulic Research 37: 789-803.
- Vermaas DA, Uijttewaal WSJ, Hoitink AJF (2007) Effect of
Heterogeneous Bed Roughness on the Conveyance Capacity
of Floodplains. Netherlands Centre for River Studies 34-35.
- Wilson CAME, Horritt MS (2002) Measuring the flow
resistance of submerged grass. Hydrological processes 16:
2589-2598.
View PDF